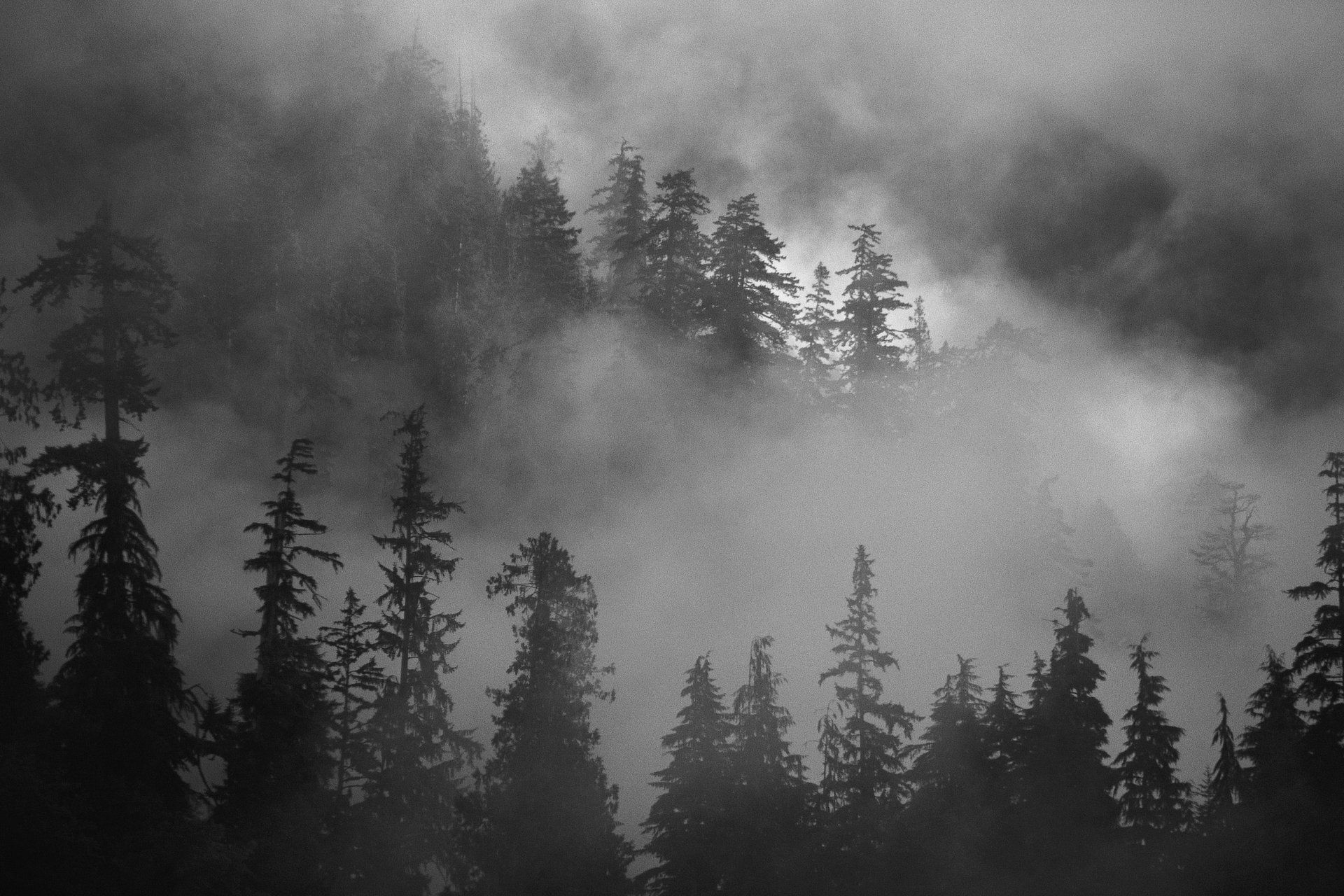
author: Kent W. Mayhew
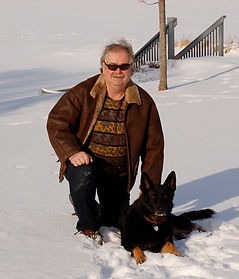
A New Thermodynamics
This book's other homepage is:
www.New-Thermodynamics.com
Abstract: Most nucleation processes involve both a phase change and a pressure change across a curved tensile layer. Therefore, both volume and pressure are relevant parameters, when determining the work involved in nucleation processes. A general equation, where the work is related to both volume and pressure change, has been derived, in part based upon a plausible explanation for latent heat changes as a function of pressure. Equations defining the work required for the nucleation of globules, liquid droplets, bubbles and cavitations, can be subsequently derived from this general equation. We also shall reanalyze the application of the Young-Laplace equations to droplets, bubbles and capillary action.
Conclusions: We realized that the Young-Laplace equation failed to consider the cohesive forces perpendicular to the tensile layer. We derived new equations for the pressure inside of a bubble (eqn 14) and a droplet (eqn 13).
Traditionally classical thermodynamics is based upon the second law with justification being purely empirical. In section 3, our analysis was an approximation, giving a plausible explanation as to why the latent heat of water decreases, as the pressure is increased. As a simple approximation we saw that the changes in latent heat with pressure may be explained by changes to the bonding energy in the vaporous state. This may help form a basis for a future satisfactory theoretical proof, which is lacking in our understanding of empirical data, as was pointed out by Sheehan 52.
Problems with previous nucleation theory became evident. Gibbs globule nucleation equation forms the basis of previous nucleation theory even though Gibbs stated that his equation is for processes “without change in entropy or volume of the system”41. Certainly, droplet and/or bubble nucleation must involve entropy and volume change.
Ultimately, traditional classical thermodynamics does not provide a general equation for calculating the work in an open system, when both pressure and volume are parameters of relevance. Equally treating pressure and volume as parameters of relevance, we start with: (29)
In terms of PV space, the work (energy) required for a process is simply: (34)
In the limit of all approaching zero, we can write a general law of thermodynamics as eqn 30. From the general law we can derive most accepted thermodynamic relations, by setting the changes of any two parameters (one on the L.H.S. and one on the R.H.S.) in eqn 30 to zero. Treating P and V equally, we derived equations for globule (eqn 1), droplet (eqn 38), and bubble (eqn 53) and nucleation.
Considering the case of a flat tensile layer, we obtained the following expected results: Eqn 38 for droplet nucleation became the latent heat of condensation Eqn 53 for bubble nucleation equation became the latent of vaporization Eqn 1 for Gibbs’s globule became zero work
We treated a cluster as an ensemble of molecules in thermal contact, prior to nucleation. We then differentiated between various types of clusters by letting g-cluster, a-cluster and l-cluster respectively represent gaseous, aqueous and liquid cluster. Now, g-clusters nucleate into droplets, a-clusters nucleate into gaseous bubbles and/or cavitations and l-clusters nucleate into vaporous bubbles. Due to lacking thermal contact, large g-clusters do not exist hence large droplets do not nucleate
We begin to understand why droplets nucleate on surfaces or around dust particles (rain). The reasoning being, the nucleation of extremely small droplets requires positive work therefore thermal contact with a heat sink is needed. As the droplet grows in size, the required work per molecule reaches a maximum at the critical radius, after which it decreases, as the droplet continues to grow. We also understand why binary mixtures, tend to form droplets with the lowest cohesive strength liquid forming the droplet’s outer tensile layer, i.e. water-alcohol droplets. The reason being, extremely small droplets require positive work to nucleate hence the probability (eqn 47) of a droplet nucleating will be greatest when the lowest cohesive strength liquid forms the droplet’s outer tensile layer.
For bubble nucleation, eqn 60 exemplifies that the work required per molecule is a decreasing function of the bubble’s radius. A reason extremely small stable bubbles do not nucleate may be: The work required per molecule is too high. The reason crevices increase the bubble nucleation rate becomes: Crevices allow clusters that are too small to nucleate homogeneously to nucleate heterogeneously because the work required per molecule is identical for equal radius cone and spherical shaped bubbles. We postulated that a given size cluster must nucleate into a given sized bubble. Hence the larger the cluster is, the higher the probability (eqn 57) of bubble nucleation must be
Boiling, which has unquestionable temperature dependence, becomes easy to understand: If a sufficiently large cluster is at/near its boiling point, then eqn 57 gives the probability that the cluster will be able to extract the work required for bubble nucleation, from its neighbors.
Research in heat transfer, which previously was not properly explained, became explainable: The various regimes for heat transfer in pool boiling. The energy required for extremely small droplets to nucleate. The increase in energy required for an extremely small droplet grow to some critical radius. The decrease in energy required for a critical radius droplet to grow in size. Why the heat transfer depends upon the number of droplets. An exceptional correlation between the theory presented here and previously unexplained phenomena was attained. The laser energy37 required to nucleate various sized water vapor bubbles, at various system pressures was plotted (Graph 8), validating our new approach to nucleation.
Cavitations were treated like any container of ideal gas hence a volume expanding force may result in a lowering of pressure within the cavitation. In so doing we would be able to explain both a cavitation forming by the expansion of a stable bubble and cavitation nucleation about a gas nuclei. Instead of gas nuclei, we envisioned a-clusters, as a dynamic ensemble of aqueous molecules, about which the liquid’s cohesive forces are a minimal. Realizing that energy is actually stored in bubbles/cavitations provides a new way of envisioning sonoluminescence (SL).
As a final thought: Even though basic equations of thermodynamics did not change, no longer being hogtied into treating as some increasing unbounded function would also simplify cosmology. Think of a blackhole as being a constant volume horizon, wherein pressure increases as more matter enters it. No wonder paradoxes occur when we apply the second law to blackholes52.
Commentary: This author realizes that one must also conider the liquid's cohesive forces perpendicular to the tensile layer, hence there are issues with using the the Young-Laplace equation to determiner the pressure within a bubble . However a mistake was made in calculating the pressure within a bubble in the paper Energetics of nucleation and this has been corrected in the paper "Pressure within a bubble revisited": Pb=1.64 (@/rb) + Patm